Computational time-of-flight diffuse optical tomography
Imaging through a strongly diffusive medium remains an outstanding challenge, in particular in applications in biological and medical imaging. Here, we propose a method based on a single-photon time-of-flight camera that allows, in combination with computational processing of the spatial and full temporal photon distribution data, imaging of an object embedded inside a strongly diffusive medium over more than 80 transport mean free paths. The technique is contactless and requires 1 s acquisition times, thus allowing Hz frame rate imaging. The imaging depth corresponds to several centimetres of human tissue and allows us to perform deep-body imaging as a proof of principle.
This is a preview of subscription content, access via your institution
Access options
Access Nature and 54 other Nature Portfolio journals
Get Nature+, our best-value online-access subscription
cancel any time
Subscribe to this journal
Receive 12 print issues and online access
206,07 € per year
only 17,17 € per issue
Buy this article
- Purchase on SpringerLink
- Instant access to full article PDF
Prices may be subject to local taxes which are calculated during checkout
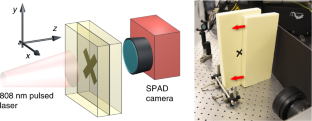
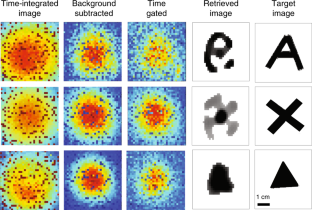
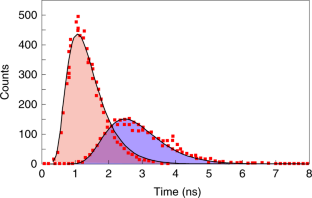
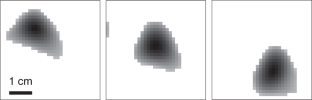
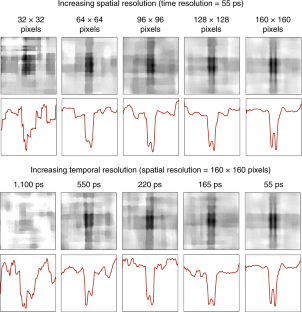
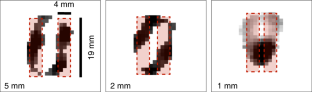
Similar content being viewed by others
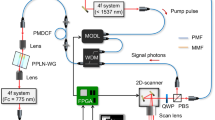
Quantum optical tomography based on time-resolved and mode-selective single-photon detection by femtosecond up-conversion
Article Open access 29 November 2023
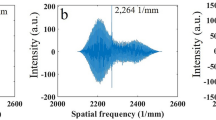
Accessing depth-resolved high spatial frequency content from the optical coherence tomography signal
Article Open access 24 August 2021
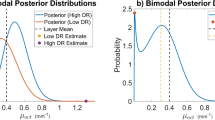
Bayesian analysis of depth resolved OCT attenuation coefficients
Article Open access 26 January 2021
Data availability
All data used in this work are available from https://doi.org/10.5525/gla.researchdata.642
Code availability
All codes used in this work are available from https://doi.org/10.5525/gla.researchdata.642
References
- Katz, O., Heidmann, P., Fink, M. & Gigan, S. Non-invasive single-shot imaging through scattering layers and around corners via speckle correlations. Nat. Photon.8, 784–790 (2014). ArticleADSGoogle Scholar
- Woo, S. et al. Three-dimensional imaging of macroscopic objects hidden behind scattering media using time-gated aperture synthesis. Opt. Express25, 32722–32731 (2017). ArticleADSGoogle Scholar
- Satat, G., Tancik, M., Gupta, O., Heshmat, B. & Raskar, R. Object classification through scattering media with deep learning on time resolved measurement. Opt. Express25, 17466–17479 (2017). ArticleADSGoogle Scholar
- Wang, L. et al. Ballistic 2-D imaging through scattering walls using an ultrafast optical Kerr gate. Science253, 769 (1991). ArticleADSGoogle Scholar
- Konecky, S. D. et al. Imaging complex structures with diffuse light. Opt. Express16, 5048 (2008). ArticleADSGoogle Scholar
- Durduran, T., Choe, R., Baker, W. B. & Yodh, A. G. Diffuse optics for tissue monitoring and tomography. Rep. Prog. Phys.73, 076701 (2010). ArticleADSGoogle Scholar
- Pifferi, A. et al. New frontiers in time-domain diffuse optics, a review. J. Biomed. Opt.21, 091310 (2016). ArticleADSGoogle Scholar
- Shi, L. & Alfano, R. R. Deep Imaging in Tissue and Biomedical Materials: Using Linear and Nonlinear Optical Methods (Pan Stanford, 2017).
- Jacques, S. L. Optical properties of biological tissues: a review. Phys. Med. Biol.58, R37–R61 (2013). ArticleADSMathSciNetGoogle Scholar
- Delpy, D. T. et al. Estimation of optical pathlength through tissue from direct time of flight measurement. Phys. Med. Biol.33, 1433–1442 (1988). ArticleGoogle Scholar
- Patterson, B., Chance, M. S. & Wilson, B. C. Time resolved reflectance and transmittance for the non-invasive measurement of tissue optical properties. Appl. Opt.28, 2331–2336 (1989). ArticleADSGoogle Scholar
- Jacques, S. L. Time resolved propagation of ultrashort laser pulses within turbid tissues. Appl. Opt.28, 2223–2229 (1989). ArticleADSGoogle Scholar
- Hebden, J. C. Evaluating the spatial resolution performance of a time-resolved optical imaging system. Med. Phys.19, 1081–1087 (1992). ArticleGoogle Scholar
- Hebden, J. C., Hall, D. J. & Delpy, D. T. The spatial resolution performance of a time-resolved optical imaging system using temporal extrapolation. Med. Phys.22, 201–208 (1995). ArticleGoogle Scholar
- Gibson, A. P. & Dehghani, A. Diffuse optical imaging. Philos. Trans. R. Soc. A367, 3055–3072 (2009). ArticleADSMathSciNetGoogle Scholar
- Berg, R., Jarlman, O. & Svanberg, S. Medical transillumination imaging using short-pulse diode lasers. Appl. Opt.32, 574–579 (1993). ArticleADSGoogle Scholar
- Grosenick, D., Wabnitz, H., Rinneberg, H. H., Moesta, K. T. & Schlag, P. M. Development of a time-domain optical mammograph and first in vivo applications. Appl. Opt.38, 2927–2943 (1999). ArticleADSGoogle Scholar
- Boas, D. A. et al. Imaging the body with diffuse optical tomography. IEEE Signal Process. Mag.18, 57–75 (2001). ArticleADSGoogle Scholar
- Torricelli, A. et al. Time domain functional NIRS imaging for human brain mapping. Neuroimage85, 28–50 (2014). ArticleGoogle Scholar
- Eggebrecht, A. T. et al. Mapping distributed brain function and networks with diffuse optical tomography. Nat. Photon.8, 448–454 (2014). ArticleADSGoogle Scholar
- Dalla Mora, A. et al. Towards next-generation time-domain diffuse optics for extreme depth penetration and sensitivity. Biomed. Opt. Express6, 1749–1760 (2015). ArticleGoogle Scholar
- Pavia, J. M., Wolf, M. & Charbon, E. Single-photon avalanche diode imagers applied to near-infrared imaging. IEEE J. Sel. Top. Quantum Electron.20, 3800908 (2014). Google Scholar
- Gibson, A. P., Hebden, J. C. & Arridge, S. R. Recent advances in diffuse optical imaging. Phys. Med. Biol.50, R1–R43 (2005). ArticleADSGoogle Scholar
- Ripoll, J., Nieto-Vesperinas, M. & Carminati, R. Spatial resolution of diffuse photon density waves. J. Opt. Soc. Am. A16, 1466 (1999). ArticleADSGoogle Scholar
- Azizi, L., Zarychta, K., Ettori, D., Tinet, E. & Tualle, J.-M. Ultimate spatial resolution with diffuse optical tomography. Opt. Express17, 12132 (2009). ArticleADSGoogle Scholar
- Satat, G., Heshmat, B., Raviv, D. & Raskar, R. All photons imaging through volumetric scattering. Sci. Rep.6, 33946 (2016). ArticleADSGoogle Scholar
- Cai, W. et al. Time-resolved optical diffusion tomographic image reconstruction in highly scattering turbid media. Proc. Natl Acad. Sci. USA93, 13561–13564 (1996). ArticleADSGoogle Scholar
- Gariepy, G. et al. Single-photon sensitive light-in-fight imaging. Nat. Commun.6, 6021 (2015). ArticleADSGoogle Scholar
- Yoo, K., Liu, F. & Alfano, R. When does the diffusion approximation fail to describe photon transport in random media? Phys. Rev. Lett.64, 2647 (1990). ArticleADSGoogle Scholar
- Wang, L. V. & Wu, H.-I. in Biomedical Optics: Principles and Imaging Ch. 8, 249–281 (Wiley, 2007).
- Jacques, S. L. Optical properties of biological tissues: a review. Phys. Med. Biol.58, 5007–5008 (2013). ArticleMathSciNetGoogle Scholar
- Gyongy, I. et al. A 256 × 256, 100-kfps, 61% fill-factor SPAD image sensor for time-resolved microscopy applications. IEEE Trans. Electron. Dev.65, 547 (2018). ArticleADSGoogle Scholar
- Combettes, P. L. & Pesquet, J.-C. in Fixed-Point Algorithms for Inverse Problems in Science and Engineering (eds Bauschke, H. H. et al.) Ch. 10, 185–212 (Springer, 2011).
- Komodakis, N. & Pesquet, J.-C. Playing with duality: an overview of recent primal-dual approaches for solving large-scale optimization problems. IEEE Signal Process. Mag.32, 31–54 (2015). ArticleADSGoogle Scholar
- Mallat, S. A. Wavelet Tour of Signal Processing 2nd edn (Academic Press, 2009).
- Rudin, L. I., Osher, S. & Fatemi, E. Nonlinear total variation based noise removal algorithms. Physica D60, 259–268 (1992). ArticleADSMathSciNetGoogle Scholar
- Berisha, S. & Nagy, J. G. in Academic Press Library in Signal Processing Vol. 4 (eds Chellappa, R. & Theodoridis, S.) 193–247 (Elsevier, 2014).
Acknowledgements
D.F. acknowledges financial support the Engineering and Physical Sciences Research Council, UK (grants EP/M006514/1 and EP/M01326X/1). Y.W. acknowledges financial support from the Engineering and Physical Sciences Research Council, UK (grants EP/M008843/1 and EP/M011089/1).
Author information
Authors and Affiliations
- School of Physics and Astronomy, University of Glasgow, Glasgow, UK Ashley Lyons & Daniele Faccio
- School of Computing Science, University of Glasgow, Glasgow, UK Francesco Tonolini
- Institute of Photonics and Quantum Sciences, Heriot-Watt University, Edinburgh, UK Alessandro Boccolini
- Institute of Sensors, Signals and System, Heriot-Watt University, Edinburgh, UK Audrey Repetti & Yves Wiaux
- Institute for Micro and Nano Systems, University of Edinburgh, Edinburgh, UK Robert Henderson
- Ashley Lyons